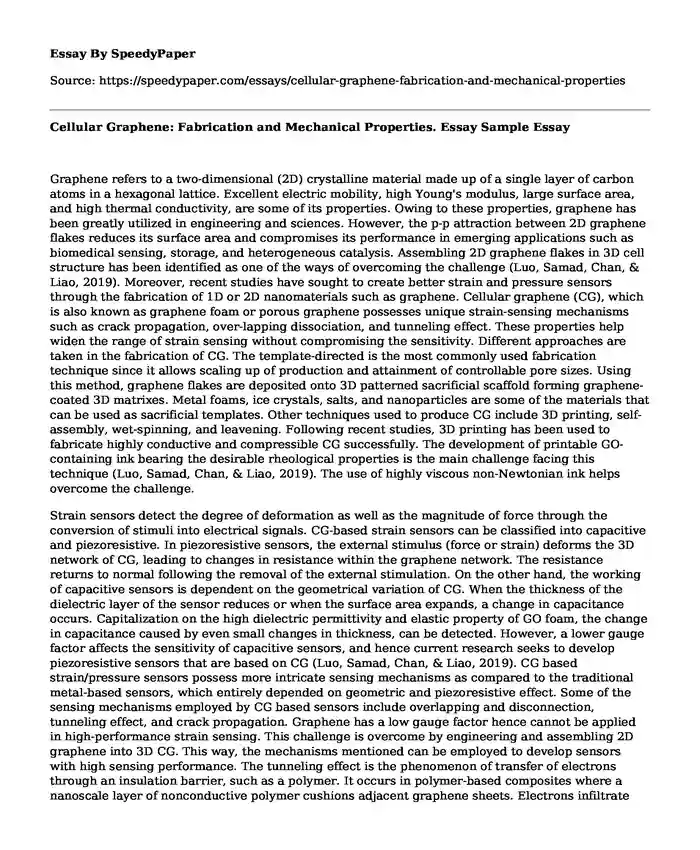
Type of paper:Â | Essay |
Categories:Â | Knowledge Engineering Chemistry |
Pages: | 4 |
Wordcount: | 1020 words |
Graphene refers to a two-dimensional (2D) crystalline material made up of a single layer of carbon atoms in a hexagonal lattice. Excellent electric mobility, high Young's modulus, large surface area, and high thermal conductivity, are some of its properties. Owing to these properties, graphene has been greatly utilized in engineering and sciences. However, the p-p attraction between 2D graphene flakes reduces its surface area and compromises its performance in emerging applications such as biomedical sensing, storage, and heterogeneous catalysis. Assembling 2D graphene flakes in 3D cell structure has been identified as one of the ways of overcoming the challenge (Luo, Samad, Chan, & Liao, 2019). Moreover, recent studies have sought to create better strain and pressure sensors through the fabrication of 1D or 2D nanomaterials such as graphene. Cellular graphene (CG), which is also known as graphene foam or porous graphene possesses unique strain-sensing mechanisms such as crack propagation, over-lapping dissociation, and tunneling effect. These properties help widen the range of strain sensing without compromising the sensitivity. Different approaches are taken in the fabrication of CG. The template-directed is the most commonly used fabrication technique since it allows scaling up of production and attainment of controllable pore sizes. Using this method, graphene flakes are deposited onto 3D patterned sacrificial scaffold forming graphene-coated 3D matrixes. Metal foams, ice crystals, salts, and nanoparticles are some of the materials that can be used as sacrificial templates. Other techniques used to produce CG include 3D printing, self-assembly, wet-spinning, and leavening. Following recent studies, 3D printing has been used to fabricate highly conductive and compressible CG successfully. The development of printable GO-containing ink bearing the desirable rheological properties is the main challenge facing this technique (Luo, Samad, Chan, & Liao, 2019). The use of highly viscous non-Newtonian ink helps overcome the challenge.
Strain sensors detect the degree of deformation as well as the magnitude of force through the conversion of stimuli into electrical signals. CG-based strain sensors can be classified into capacitive and piezoresistive. In piezoresistive sensors, the external stimulus (force or strain) deforms the 3D network of CG, leading to changes in resistance within the graphene network. The resistance returns to normal following the removal of the external stimulation. On the other hand, the working of capacitive sensors is dependent on the geometrical variation of CG. When the thickness of the dielectric layer of the sensor reduces or when the surface area expands, a change in capacitance occurs. Capitalization on the high dielectric permittivity and elastic property of GO foam, the change in capacitance caused by even small changes in thickness, can be detected. However, a lower gauge factor affects the sensitivity of capacitive sensors, and hence current research seeks to develop piezoresistive sensors that are based on CG (Luo, Samad, Chan, & Liao, 2019). CG based strain/pressure sensors possess more intricate sensing mechanisms as compared to the traditional metal-based sensors, which entirely depended on geometric and piezoresistive effect. Some of the sensing mechanisms employed by CG based sensors include overlapping and disconnection, tunneling effect, and crack propagation. Graphene has a low gauge factor hence cannot be applied in high-performance strain sensing. This challenge is overcome by engineering and assembling 2D graphene into 3D CG. This way, the mechanisms mentioned can be employed to develop sensors with high sensing performance. The tunneling effect is the phenomenon of transfer of electrons through an insulation barrier, such as a polymer. It occurs in polymer-based composites where a nanoscale layer of nonconductive polymer cushions adjacent graphene sheets. Electrons infiltrate the thin insulating layer and form quantum tunneling junctions, which in turn lead to the formation of conductive percolating networks. Using the Simmon's theory, tunneling effect is affected by various factors such as the electrical potential difference, the cross-section area of the tunnel, the tunneling current density, height of the energy barrier, electron charge, among others (Luo, Samad, Chan, & Liao, 2019). When CG is built up using overlapping graphene cell walls/struts, the electrical resistance is dependent on the contact condition between the adjacent graphene cell walls. This forms the basis of the overlapping-disconnecting mechanism. When the graphene framework is stretched, sliding between adjacent graphene struts occurs, reducing contact area, and hence electrical resistance increases. Following the release of the applied loading, the graphene sheets go back to their regular stress-free positions. The reversible overlapping and disconnecting behavior of the graphene cell walls causes variation in resistance. Studies have shown that defect-concentrated areas in the structure are usually the locations of the microscopic crack formation when CG is deformed. The crack propagation mechanism is based on this phenomenon. When deformation increases, the separation between overlapping flakes increases, leading to an increase in density and size of the microcracks, which then increases electrical resistance. On the removal of external loading, the microcracks reconnect, and the resistance goes back to the original state (Luo, Samad, Chan, & Liao, 2019).
Nanomaterials are preferred over the conventional materials in sensor applications for various reasons. Modern sensor applications such as in wearables and stretchables are required to have high sensitivity and an extended dynamic range. The brittleness and weight of conventional materials, therefore, makes them unsuitable. In their place, nanomaterials are used since they are light in weight and stretchable. The higher sensitivity of nanomaterials as compared to conventional materials is arguably the most significant advantage of nanomaterials. It has led to enhanced performance, and the applications of nanomaterials in sensors has increased. Nanomaterials also have a larger surface area than conventional materials, a factor that enhances their performance. Moreover, nanomaterials have superior mechanical properties, which allow further processing as well as utilization in different fields. The processing helps overcame any flaws associated with the nanomaterials. For instance, while compressible honeycomb-like CGs can be used to make pressure sensors, they have low sensitivity and hence cannot detect subtle changes in pressure. However, further processing can help overcome this challenge. The introduction of nanosized air bubbles within the honeycomb-like CG results in a porous graphene film whose sensitivity is greatly enhanced (Luo, Samad, Chan, & Liao, 2019).
References
Luo, S., Samad, Y. A., Chan, V., & Liao, K. (2019). Cellular Graphene: Fabrication, Mechanical Properties, and Strain-Sensing Applications. Matter, 1(5), 1148-1202.
Cite this page
Cellular Graphene: Fabrication and Mechanical Properties. Essay Sample. (2023, Mar 12). Retrieved from https://speedypaper.com/essays/cellular-graphene-fabrication-and-mechanical-properties
Request Removal
If you are the original author of this essay and no longer wish to have it published on the SpeedyPaper website, please click below to request its removal:
- Essay Example about Community Gatekeeper Identification
- Industrial Design - Personal Statement Essay Example
- Free Essay Dedicted to David Hockney and His Connection to Modernism Movement
- Compare and Contrast Essay Samle on the Articles of Confederation and the Sonstitution
- Essay Example on Titanic Technology and Security
- Free Essay on Reducing HCV Prevalence in Incarcerated Population
- Career Essay Example: Accountant - Financial Advisor
Popular categories